Germaine Gogel
Department/Office Information
ChemistryContact
ggogel@colgate.eduBS (1974), Purdue University; MS (1976), PhD (1979), Northwestern University
Metabolic activity in photosynthetic bacteria; Structure/function relationships in chlorophyll-binding proteins.
My recent research projects have involved investigating changes in the metabolic activity of photosynthetic bacteria grown with herbicides in the growth media. Photosynthetic bacteria are among many microbes that are a part of the natural soil and water microbiome. My research students have used glyphosate (the primary ingredient in Roundup™) and atrazine to inhibit bacterial growth in the laboratory and have investigated alterations in metabolic pathways induced by exposure to the herbicides.
Currently I am winding down my research activities in anticipation of retirement. I am looking forward to teaching my CORE course on Native American Medicine and the General Chemistry course in Fall 2019 and will then lead the Fall 2020 NIH Study Group as my final study abroad semester.
- CHEM 101 and 102: General Chemistry I and II
- CHEM 353: Proteins and Nucleic Acids
- CHEM 385: Biophysical Chemistry Methods
- CHEM 452: Metabolic Chemistry
- CHEM 454: Bioenergetics
- CHEM 468: Medicinal Chemistry
- CORE 136S: Critical Analysis of Health Issues: Cancer
- CORE 177S: Critical Analysis of Health Issues: Native American Medicine
- ENST 391Y (or NASC 391Y): Energy and Environment in Wales and the UK
- UNST 335Y: Health and Healing Practices in Australia: Traditional Healing and Post-Colonial Adaptations
- UNST 330Y/AHUM 330Y: Health and Healing Practices in Wales: From Ancient Traditions to Modern Medicine
Biochemistry, proteins, metabolism
- Postdoctoral Research Associate at Cornell University (1980) with Aaron Lewis, Applied & Engineering Physics
- Visiting Research Associate at University College London (1985) with Jim Barber in Pure & Applied Biology and at The Medical College of Virginia (1989) with George DeVries in the Department of Biochemistry
- Special Volunteer at The National Institutes of Health (2001) with Florence Davidson in the National Cancer Institute
- Study group leader for Study Abroad semesters at Cardiff University, Cardiff, Wales (Spring 1988, 2003, 2017), The National Institutes of Health (Fall 2001, 2011, upcoming 2020) in Bethesda, MD, and the University of Wollongong, Wollongong, Australia (Spring 2013)
G. E. Gogel, “Guided inquiry sessions to supplement a large lecture course: aiding and retaining struggling students,” 23th Biennial Conf. on Chemical Educ. Proceedings (2014), in press
G. E. Gogel, “Using guided inquiry sessions to supplement a traditional general chemistry lecture.” 20th Biennial Conf. on Chemical Educ. Proceedings (2008), 323.
G. E. Gogel, H. March*, A. Friedrich* and P. Schwartz*, “Chemical Modification of Tryptophans and Lysines in the B880 Light-Harvesting Protein of Rhodospirillum rubrum,” Progress in Photosynthesis Research (1987), 2, 29-32.
Gogel, G.E., N.J. Russell, and J. Harwood, “Changes in Fatty Acid Synthesis During Temperature Adaptation in Rhodobacter sphaeroides,” Biochemical Society Transactions (1989), 17, 689-690.
P. A. Millner, G. Gogel and J. Barber, “Investigation of the Spatial Relationships between Photosystem II Polypeptides by Reversible Cross-Linking and Diagonal Electrophoresis,” Photosyn. Res. (1987), 13, 185-198.
G. E. Gogel, M. Michalski*, H. March*, S. Coyle* and L. Gentile*, “Covalent Modification of Lysines of the B880 Light-Harvesting Protein of Rhodospirillum rubrum,” Biochemistry (1986), 25, 7105-7109.
G. E. Gogel and P.A. Millner, “Chemical Cross-Linking of Photosystem II Particles and Removal of Polypeptides by Salt and Alkaline Tris Washing,” Biochem. Soc. Trans. (1986), 14, 43-44.
(Asterisks indicate undergraduate student co-authors who conducted their research in collaboration with a faculty member at Colgate.)
NSF S-STEM Grant (PI) 2007-14, NIH Senior Postdoctoral Fellowship 1989, NIH Academic Research Enhancement Award 1988-91, Research Corporation Grant 1983, NIH Postdoctoral Research Fellowship 1980
Recent Research Projects:
My research probes the biochemistry of photosynthetic bacteria, especially the functions of their membranes, and in particular, the proteins associated with their membranes. The earliest forms of photosynthetic bacteria are anoxygenic and evolved long before oxygenic (oxygen producing) organisms (Blankenship 2010). One of the six types of simple photosynthetic prokaryotes is purple non-sulfur bacteria. Some of my research projects utilize Rhodospirillum rubrum, a gram-negative purple, non-sulfur photosynthetic bacteria found in ponds, lakes, streams, and standing water (Reslewic et al. 2005) and also in mud and sewage (Madigan et al. 2000). These bacteria are facilitative anaerobes that are capable of growing aerobically (non-photosynthetically), consuming carbon compounds in an oxygen atmosphere, or anaerobically by using light as its energy source and fixing carbon dioxide, i.e. photosynthetic growth (Madigan et al. 2000).
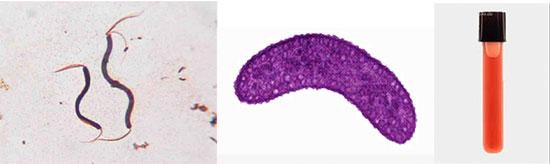
Glyphosate (chemical name N-[phosphonomethyl]glycine) is the primary chemical ingredient in the non-specific herbicide Roundup™. Many genetically modified organisms (GMOs) have been engineered by Monsanto to be resistant to glyphosate. About 250 million pounds of Roundup™ were used to kill weeds in fields of GMO crops in the US and about 26 million pounds were used on lawns and gardens by individuals, industries and municipalities in 2014. (National Agricultural Statistics Service (NASS) 2014) In plants, glyphosate acts by inhibiting the enzyme 5-enolpyruvylshikimic acid-3-phosphate (EPSP) synthase and disrupts the shikimic acid pathway (Duke and Powles 2008). The EPSP synthase deficiency results in reduction of the aromatic amino acids necessary for protein synthesis and plant growth (Glyphosate Technical Fact Sheet; Banaszkiewicz et al. 2012). The EPSP synthase enzyme inhibited by glyphosate is unique to plants, algae, and some microorganisms and is not present in mammals (Duke and Powles 2008).
Herbicides which contain glyphosate are applied by spraying an aqueous solution. Glyphosate is absorbed by the plant through the leaves and roots and is also deposited in the soil and found in aqueous run-off (Banaszkiewicz et al. 2012). Glyphosate adsorbs to soil particles, and a few species of soil micro-organisms, but not all, have been shown to degrade glyphosate (Huang et al. 2005). Plants can slowly degrade a limited amount of glyphosate using an oxidative pathway, some extrusion of glyphosate occurs through the plant roots, and glyphosate and its degradation products also accumulate in glyphosate-resistant plants (Duke 2011; Reddy et al. 2008).
We have studied the inhibitory effects of glyphosate on a photosynthetic bacteria commonly found in streams and ponds, Rhodospirillum rubrum. Also, this species is capable of utilizing some organic compounds as a carbon source, and we have explored the ability of the bacteria to degrade glyphosate to harmless compounds.
Banaszkiewicz, T.; andWysocki, K. "Application of White Mustard (Sinapis alba) Biotest in the Assessment of Environmental Contamination by Glyphosate." Polish Journal of Environmental Studies 2012, 21.5, 1161-66.
Blankenship, R. E., Early Evolution of Photosynthesis. Plant Physiology 2010, 154, 434-438.
Druart, C.,; Delhomme, O.; De Vaufleury, A.; Ntcho, E.; Millet, M. “Optimization of Extraction Procedure and Chromatographic Separation of Glyphosate and Aminomethylphosphonic Acid in Soil.” Analytical and Bioanalytical Chemistry 2011, 399, 1725-32.
Duke, S. O. “Glyphosate Degradation in Glyphosate-Resistant and -Susceptible Crops and Weeds.” J. Agric. Food Chem. 2011, 59, 5835-5841.
Duke, S. O.; Powles, S. “Glyphosate: a once-in-a-century herbicide: Mini-review.” Pest Manag. Sci. 2008, 64, 319–325.
US EPA 2007 Pesticide Market Estimates: Agriculture, Home and Garden.
Falkowski, P. G. “The biological and geological contingencies for the rise of oxygen on Earth.” Photosynth. Res. 2011, 107, 7-10.
Glyphosate Technical Fact Sheet. National Pesticide Information Center. N.p., n.d. Web. 12 Dec. 2013.
Huang, J.; Su, Z.; Xu, Y; “The Evolution of Microbial Phosphonate Degradative Pathways.” J. Mol. Evol. 2005, 61, 682-690.
Madigan, M. T.; Martinko, J. M.; Parker, J. Brock Biology of Microorganisms. 9th ed. Upper Saddle River: Prentice Hall, 2000.
National Agricultural Statistics Service (2014) U.S. soybean industry: glyphosate effectiveness declines, NASS highlights No. 2014-1.
Reddy, K. M.; Rimando, S. M.; Duke, S. O.; Nandula, V. K. “Aminomethylphosphonic acid accumulation in plant species treated with glyphosate.” J. Agric. Food Chem. 2008, 56, 2125-2130.
Reslewic, S., et al. “Whole Genome Shotgun Optical Mapping of Rhodospirillum rubrum.” Applied and Environmental Microbiology 2005, 71, 5511-22.